Of Computers and Codes
The Solar Corona
Heliospheric Structure
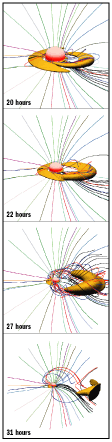 |
Figure 1.
CME in 3-D
The evolution of a CME eruption in a three-dimensional MHD simulation, showing an isodensity surface of the plasma blob and the magnetic field lines. The CME is being ejected from the simulation volume in the last frame. |
he Sun’s activity waxes and wanes on an eleven-year cycle. Sunspots, flares, and coronal mass ejections (CMEs)–spectacular eruptions of huge blobs of magnetized plasma from the Sun’s outer atmosphere into interplanetary space–are less frequent at solar minimum. But with the Sun now close to maximum activity, they are commonplace; they give rise to "space weather" that can affect events on and near Earth. A group of researchers at Science Applications International Corporation (SAIC) is using NPACI’s supercomputers to model solar active regions and magnetic fields, the triggering of flares, the eruption of prominences and CMEs, and variations in the structures of the corona and the solar wind during active, quiet, and intermediate phases of the Sun’s cycle. Their results are giving scientists a better understanding of the workings of our star and of the space plasma environment, and are helping to predict the space weather.
Researchers at SAIC have developed sophisticated code packages to model the activity of the Sun and of the heliosphere, that immense volume of plasma that contains our solar system, the solar wind, and the entire solar magnetic field. The space scientists’ codes model in three dimensions the initiation of solar activity and the evolution of solar active regions, the outer structure of the Sun’s atmosphere (the corona), and the large-scale structure of the heliosphere. Their solar and heliospheric simulations are the result of more than 10 years of development and application of 3-D magnetohydrodynamic (MHD) simulation algorithms. These in turn were adaptations of earlier development efforts at SAIC to model MHD effects for fusion applications.
Of the phenomena under study, coronal mass ejections have the greatest effects on the immediate space environment and on life on Earth. The enormous clouds of plasma travel outward from the Sun; if they happen to collide with our planet’s magnetic field they can cause geomagnetic storms, which can damage orbiting satellites, disrupt radio communications, and even damage electrical power distribution grids on Earth. And the spectacular auroras of the past several months, which have been visible in the night sky as far south as Arizona, result from CME plasma clouds interacting with Earth’s magnetosphere and the highest reaches of the upper atmosphere.
"We are researching fundamental problems and using our results in the prediction of structure in the corona and heliosphere," said senior research scientist Jon Linker, the principal investigator of the NPACI allocation. "By self-consistently modeling the flow of plasma from the Sun’s surface to beyond Earth’s orbit we can address important space weather questions." The SAIC group’s work is supported by NASA’s Sun-Earth Connections Theory and Supporting Research and Technology Programs, and by an NSF-sponsored consortium, headed by Boston University, for developing an integrated space weather model.
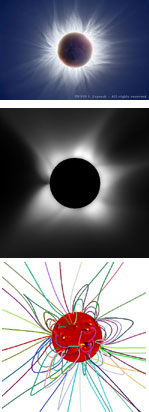 |
Figure 2. Solar Eclipse: Model vs. Reality
The image at left is a composite of 22 separate photographs of the total solar eclipse of August 11, 1999; they were digitally processed and combined to enhance subtle features and structure in the corona. Image copyright 1999 by Fred Espenak (www.MrEclipse.com). The center image shows the predicted polarization brightness of the corona as simulated by the MAS 3-D MHD model. The magnetic field lines from the model are depicted at right.
Copyright 1999 by Fred Espenak, courtesy of www.MrEclipse.com/MrEclipse.html.
|
Of Computers and Codes
"Using NPACI’s resources, we can model a variety of complex plasma processes at the Sun and in the solar wind," said senior research scientist Zoran Mikic´. "Our research helps to interpret observations of active regions and to understand the role of magnetic fields in structuring the solar corona. It allows us to place quantitative constraints on the mechanisms that heat the solar corona, and to explore the role of photospheric shear and emerging flux in solar activity. The coronal magnetic field is of central importance to understanding and predicting solar activity."
"We’ve been using NPACI allocations on the Cray T90, the Cray T3E, and Blue Horizon at SDSC, and additionally on machines at the National Energy Research Supercomputer Center," Linker said. "Intermediate heliospheric structure calculations with MAS require up to a million grid points, and are best suited for the T90. We are developing MASP, a parallel version of the code, to perform considerably larger simulations on Blue Horizon. These simulations, using very large meshes–tens of millions of grid points–are intended to capture fine-scale features of coronal phenomena, such as prominences, in a realistic simulation of the entire corona. We hope this will demonstrate that the fine-scale features seen in spacecraft observations of the Sun are accessible to computational description."
To extend the capabilities of their solar simulations, the group is developing MH4D, a new parallel algorithm to solve the time-dependent MHD equations on an unstructured tetrahedral grid of arbitrary shape and connectivity. "MH4D requires more computation time per cell but allows a much more flexible distribution of cells," said senior research scientist Dalton Schnack. Most of the results presented here are from simulations run on NPACI’s Cray T90 at SDSC, and were presented at the December 2000 meeting of the American Geophysical Union.
The Solar Corona
"A continuing focus is the structure of the large-scale solar corona and its large-scale disturbances–coronal mass ejections," said research scientist Roberto Lionello. "For this we use the MAS and MASP codes, which solve the MHD equations in spherical coordinates." Figure 1 shows one such CME simulation.
The group’s research on solar active regions is based on using theoretical models to study the mechanism that heats the corona, the triggering of solar flares, and the eruption of prominences. Events at the Sun’s surface affect the space around it, eventually to the edge of the solar system.
The state of the corona is predicted from a theoretical model based on the MHD equations. The magnetic field at the photosphere (the Sun’s visible surface) can be measured from ground-based observations. This information is used to solve the 3-D MHD equations to steady state in the corona, balancing the interaction of the solar wind with the coronal plasma to predict the magnetic field, density, temperature, and flow velocity of the coronal plasma.
The pearly glow of the corona during a total solar eclipse is sunlight scattered off electrons in the coronal plasma. To test their models against reality, the researchers generate synthetic images from their simulations (Figure 2) and compare them to images recorded during eclipses and obtained from ground and satellite-based coronagraphs.
On June 21, 2001, a total eclipse of the Sun will be visible from parts of southern Africa and Madagascar. In mid-May the SAIC researchers began simulating the solar corona in anticipation of this event, and they have continued to update their prediction using more recent photospheric magnetic field data. Their latest prediction of the corona’s appearance during the eclipse is on the group’s Web site; images taken on the day of the eclipse also will be posted when available.
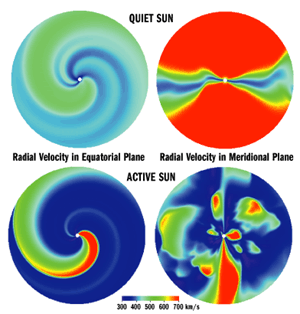 |
Figure 3. The Distant Wind
Reconstructions of the solar wind at low solar activity in August-September 1996 (top) and high solar activity in August-September 1999 (bottom) in a volume five times the diameter of Earth‚s orbit. During high solar activity, the velocity structures are much more complex. |
Heliospheric Structure
The group also has been using MAS to model the large-scale structure of the heliosphere at various stages of the solar cycle, to study the configuration of the magnetic field at solar maximum, and to interpret solar wind observations from the SOHO, Ulysses, and Wind spacecraft.
"We have undertaken simulations of the inner heliosphere out to 5 AU–roughly the orbit of Jupiter–to explore the changes that occur between solar minimum and maximum," Linker said (Figure3). "We can address several questions: How does the heliosphere’s structure change during a solar cycle? What is the origin of the slow and variable component of the solar wind? We plan to develop heliospheric solutions by using coronal calculations to specify the inner boundary conditions."
"We are beginning to compare our results with plasma and magnetic field measurements from the ACE, Wind, and Ulysses spacecraft," research scientist Pete Riley said. "For the first time we can simulate the plasma and magnetic field properties of the heliosphere for specific time periods of interest. The model allows us to interpret single-point spacecraft measurements from a global perspective, and provides a contextual basis with which to correlate spacecraft measurements and solar observations."
-MG 
|
RESEARCHERS
Jon A. Linker,
Roberto Lionell,
Zoran Mikic´,
Pete Riley,
Dalton Schnack
Science Applications International Corporation |